Smallholder farmers in the global South mostly use traditional crop varieties, which can be vulnerable to climate impacts such as droughts, floods or also diseases. In order to improve the resilience of crops to climatic shocks and to raise yields, improved crop varieties are bred from traditional varieties. The process of breeding is lengthy and costly, but once better varieties are released and used, they can substantially improve agricultural yields and resilience, depending on their specific characteristics. As such, breeding improved varieties is an institution-led approach, since it requires resources and time, which smallholder farmers most often do not have.
Improved crop varieties can offer a number of interesting development co-benefits, especially linked to increased agricultural production and income. However, they are expensive to develop: a multitude of factors will determine prices of the seeds, such as demand, scale of adoption, and – for farmers – potential government subsidies
A number of improved crop varieties already exist or are being developed in Ghana, for instance for maize, rice, cassava and cocoa, with sought-after properties being drought resistance, flood resistance and achievement of high yields. Maize appears to be the focus of breeding efforts in Ghana, as several publications (e.g. Alhassan, Salifu & Adebanji, 2016; Danso-Abbeam et al., 2017) and the number of active breeders for maize (10 out of 26) confirm (Mabaya et al., 2017).
An analysis conducted using the biophysical crop model APSIM shows that improved maize varieties indeed hold large potential for increasing yields under climate change (Figure 3). However, the size of the effect and sometimes even direction depends on the location, with the impact in different districts in Ghana differing considerably. Compared to no adaptation and other agronomic adaptation measures such as applying manure or delaying the sowing date, a carefully selected improved variety may indeed have a larger impact on increasing maize yields.
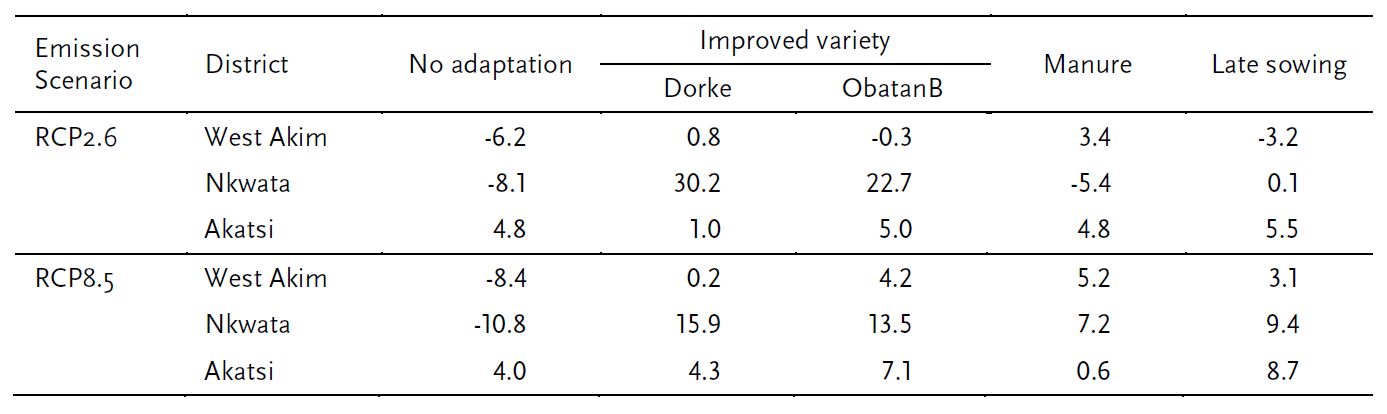
Another analysis conducted with machine-learning based crop suitability models shows how generally the effect of agronomic adaptation strategies varies considerably across crops. While maize and cassava appear to benefit from many agronomic adaptation strategies under climate change, for sorghum this is much more the case under the high-emission climate scenario (RCP8.5) and for groundnut, none of the adaptation strategies proposed has a positive effect.
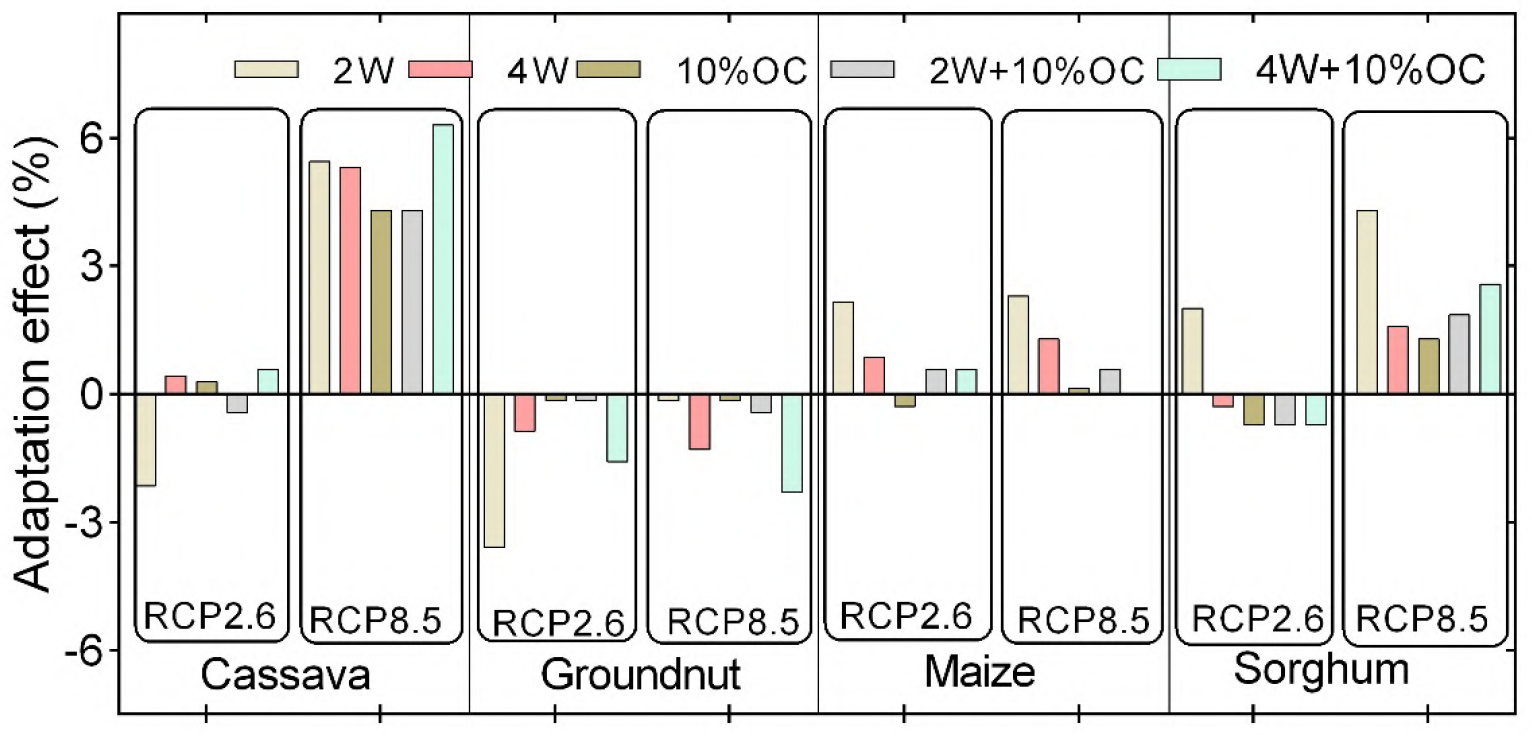
Agronomic adaptation strategies and the use of improved seeds should thus be carefully evaluated for each specific region, crop and climate impact scenario. Nonetheless, improved crop varieties are seen as a transformative tool for buffering climate impacts in Ghanaian agriculture. Breeding is costly and time intensive, but where improved varieties already exist, they can contribute importantly to a higher agricultural output.
References
- Alhassan, A., Salifu, H., & Adebanji, A. O., (2016). Discriminant analysis of farmers’ adoption of improved maize varieties in Wa Municipality, Upper West Region of Ghana. SpringerPlus, 5(1).
- Danso-Abbeam, G., Bosiako, J. A., Ehiakpor, D. S., & Mabe, F. N., (2017). Adoption of improved maize variety among farm households in the northern region of Ghana. Cogent Economics and Finance, 5(1), 1–14.
- Mabaya, E., Adzivor, S. Y., Wobil, J., & Mugoya, M., (2017). Ghana Brief 2017 – The African Seed Access Index, (December).